Tailored Strength of ultrafine-grained and nanostructured materials for Dynamic Loading Applications
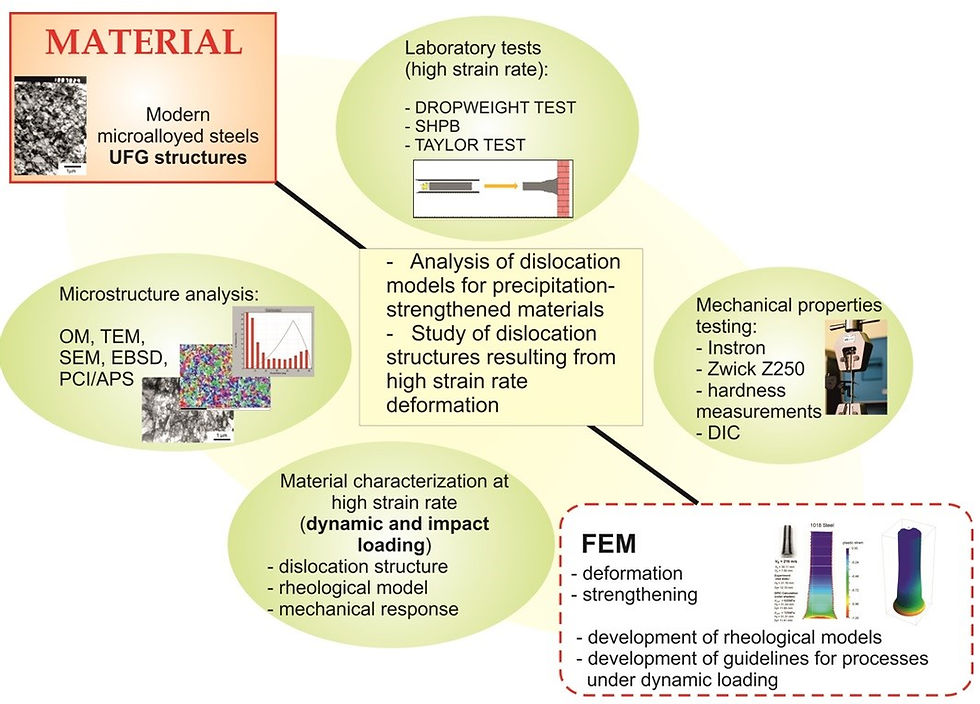
The main advantage of grain refinement down to ultrafine-grained (UFG) regime is a significant increase in strength and change in physical properties. This opens up the posibilities for their broader application in innovative industries such as transport, energy and bioengineering. UFG materials exhibit a very high strength not possible to obtain by other strengthening mechanisms. A number of applications subject also UFG and nanostructured materials to the extreme environment of high stress, dynamic loading, fatigue. For many of these applications it is desirable to not only understand failure but also to predict it. Currently, the ability to do this is limited due to the lack of understanding of the microstructural evolution sustained by materials under dynamic loading conditions and physical representation of this understanding within high rate plasticity models. For example, modern microalloyed ultrafine-grained (UFG) steels are high strength versions of their ancestors. To date, most often the evaluation of the usefulness, and therefore, selection of these structural materials for applications takes place through supported by experimental results comparison of quasi-static and dynamic analysis. As the number of available, advanced high-strength metallic materials possibilities increases due to advancements in processing (for example advanced thermomechanical processing ATP or severe plastic deformation SPD), experimental comparisons alone are not sufficient for determination of the most ideal microstructures for specific applications. Fortunately, today computer simulation capabilities and computing power is robust enough to begin to tackle the difficult problem of predicting the mechanical response of these materials under both quasi-static and dynamic loading conditions in a microstructurally aware manner. However, to do this, a physically based theory that links these small scale, microalloyed microstructures to mechanical response must be developed. Thus; The main objective of this project is two-fold: (1) improve the knowledge of the strengthening mechanisms that operate in the UFG and nanostructured metals and alloys subjected to dynamic loading conditions and (2) based on this improved knowledge, propose a microstructurally aware constitutive description between basic parameters of loading (stress state and strain rate) and mechanical behavior of representative, modern, UFG high strength structural materials.
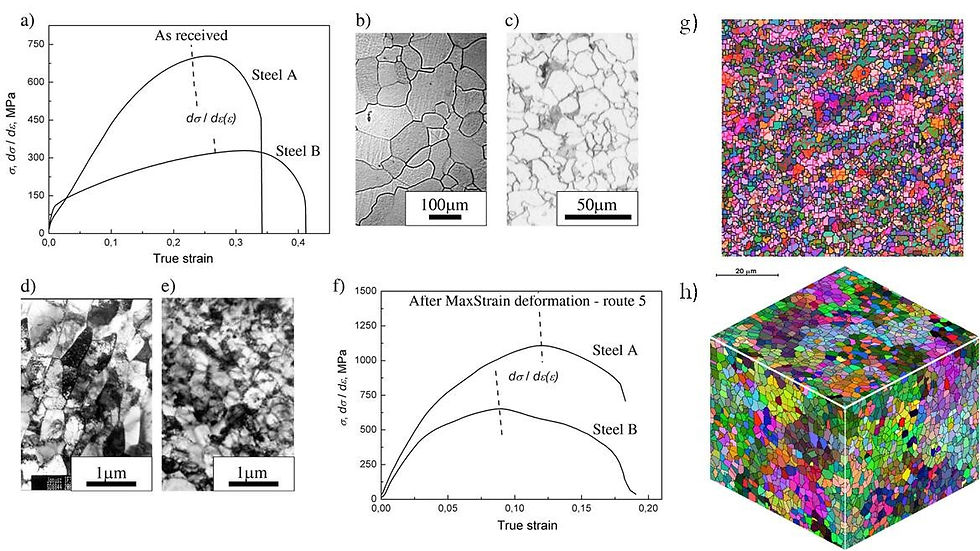
We will study the dynamic work-hardening behavior as a function of solute atoms and fine-scale, second-phase particles in the UFG and nano-structures. This knowledge of the microstructural and substructural evolution during deformation will allow us to build new constitutive models for predicting the behavior of modern, UFG and nanostructured high strength structural materials under dynamic loading conditions. The dynamic behavior of materials has become an important component to the widespread use of many structural materials. UFG materials have already passed their first tests for industrial maturity – currently available technologies allow obtain them in relatively large quantities (in the form of plates, rods and wires). Unfortunately, while the mechanical response of many metals and alloys is well understood under more conventional loading rates, a robust knowledge of the same materials under dynamic conditions is not so well understood. Previously, this has been true because of limitations in experimental and diagnostic techniques with regard to examining in-situ deformation and damage processes. Recently, new experimental and in-situ diagnostic techniques, coupled with advanced microstructure characterization techniques, are starting to allow for the detailed study of dynamic deformation and its linkage to microstructure. Moreover, these experimental advancements have been accompanied by the radical changes in analytical capabilities enabled by computers. Thus, the weight of large-scale computations has given an amazing predictive capability to the field and an ability to incorporate large data sets collected by modern experiments into mathematical models. Material model development - in general, and ultrafine-grained – in particular, is largely dependent on continuum scale phenomena and ignores the rich multiscale physical and chemical phenomena that are responsible for the macro-scale plastic deformation response of a polycrystalline metal. The substantial complexity of these phenomena, which occur through the evolution of microstructure and texture in response to dynamic loading, presents formidable challenges to theoretical model development of plastic deformation. While the experimental community has continued to push forward to identify the deterministic links between materials processing, microstructure, and dynamic properties, the primary challenge for computer modeling has been a physics-based, rheological model for multiphase and alloyed metals under dynamic loading conditions. Such a model is exactly what is desirable for modern, high strength steels, whose properties are manipulated through the use of micro-alloying, strong grain refinement and partitioning of strength amongst multiple phases. The development of this type of model can be done only by utilization of multi-scale analysis, that will be described in details in the next paragraphs. One might think that the largest application of dynamically loaded materials is in military affairs. But this may be an unfair judgment. For instance, just examine the application of a shape charge – an application that relies on the utility of an explosively loaded plate of metal. Over 90% of shape charges are employed in the oil, gas and demolition industries. The utility of these components has been found to rely heavily on microstructure and processing of the material, but detailed prediction of this linkage has not been explored. In addition to the application of shape charges, dynamic deformation of materials is one of the seminal aspects in studying and improving the crashworthiness of transportation systems.
Realization of the proposed project will manifest in a process-aware understanding of the influence of strain rate on changes in properties of finished product. In consequence it will be possible to design the new ultrafine-grained materials with given sensitivity to strain rate, respecting the process parameters as well as the strengthening models.